A new publication from Dr. Josef Penninger’s lab in the Life Sciences Institute (LSI) at UBC, and collaborators in France, Austria, and the UK, reports a major advance in the organoid field – the development of a robust microfluidic platform to vascularize biological tissues on-chip. The research, published in Nature Communications, will enable long-term culture of three-dimensional organoids, spheroids, tumoroids, or tissue explants, and enhance their growth, maturation, and function.
Organoids are 3D tissues made in the lab using stem cells or tissue cells as starting material, which in the presence of a supportive environment (extracellular matrix, growth factors), self-organize into a structure that mimics the function of real organs. Organoids have applications in disease research and drug discovery, as well as potential for cell therapy and regenerative medicine.
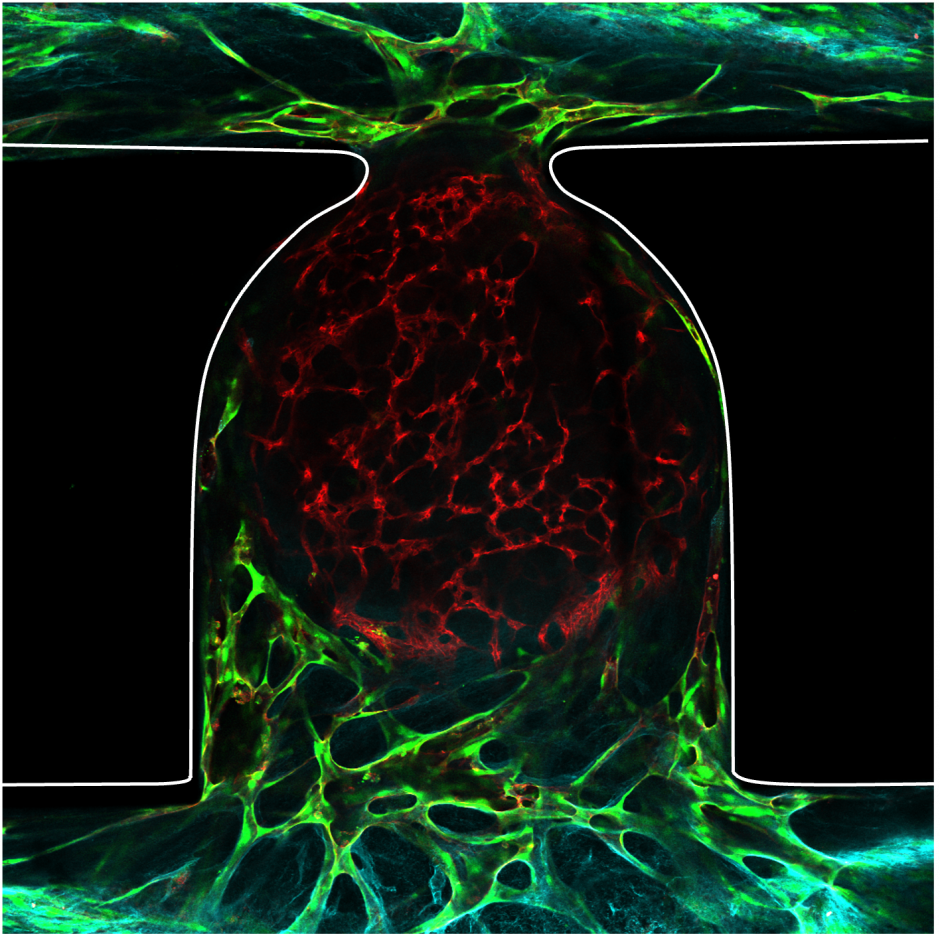
The following is a Q&A with Dr. Clément Quintard, a postdoctoral fellow in Dr. Penninger’s lab at UBC, and lead author on the study.
Q. What is the significance of this study?
CQ: By integrating microfluidics with stem cell technology, this study advances our ability to create more realistic biological models. The use of microfluidics provides a way to flow substances and cells through the stem cell-derived organoid’s own vasculature. This development opens up possibilities for enhancing the growth of organoids and introduces the potential to incorporate immune cells, a significant step for the field.
Q. What are the major challenges in the field?
CQ: A key obstacle in organoid research is their lack of an internal vascular network, which is essential for the long-term viability and function of these 3D tissues. While real organs are sustained by a complex system of blood vessels that supply oxygen and nutrients and remove waste, lab-grown organoids currently lack this crucial support. This deficiency restricts their size (with most unable to grow beyond a few millimeters) and longevity due to the inability to maintain a proper internal environment. As organoids grow, cells in the interior can become deprived of nutrients and oxygen, leading to cell death and an inability to mimic organ function accurately over long periods.
Q. How will this work help overcome these challenges?
CQ: We have developed a system that simulates the blood vessel network’s essential functions in a controlled, on-chip environment. The microfluidic platform allows for the precise delivery of nutrients and oxygen while also facilitating the removal of waste products. This was achieved by creating micro-scale channels within the chip that are capable of perfusing the organoids with a flow of medium, much like blood flows through vessels. This innovation not only addresses the diffusion barriers that previously restricted organoid growth but also paves the way for creating larger, more biologically complex organoids that can survive longer and potentially reflect the intricate workings of actual human tissues more accurately.
Q. What does the future hold for this research?
CQ: Looking forward, the research team aims to further refine the microfluidic platform to accommodate a wider range of organoid types and to enable the integration of multiple organ systems on a single chip, opening the door to more sophisticated models of human physiology. This could include the development of “human-on-a-chip” systems that replicate the interactions between different organ types, enhancing our ability to study systemic diseases and multi-organ responses to drugs.
Additionally, the team may focus on scaling up the technology for high-throughput applications in drug screening, or customizing the platform for specific diseases to create more accurate models for research and therapeutic development.
The ultimate goal is to bridge the gap between in vitro studies and clinical realities, contributing to more predictive models of human biology and accelerating the path from laboratory research to clinical solutions.
About the Researchers:
Dr. Clément Quintard, is a postdoctoral fellow in the LSI at UBC. His research aims at the convergence of stem cell biology and bioengineering, with a particular focus on providing a functional vasculature to organoid models. Dr Quintard obtained his PhD in 2022 in Biotechnology at CEA Grenoble, France.
Dr. Josef Penninger is a Professor in Medical Genetics at UBC, Canada 150 Research Chair in Functional Genetics, Former LSI Director (2018-2023), and is currently Director of the Helmholtz Centre for Infection Research (HZI) in Germany. Dr. Penninger developed the first self-organizing 3D human blood vessel organoids from embryonic stem cells (Nature, 2019), which he used to model diabetic vasculopathy.
Dr. Xavier Gidrol is the Director of the Biomics laboratory and the “Large-Scale Biology unit” (CEA – Inserm – UGA) at CEA Grenoble in France. His research is focused on microsystems for cell biology, specifically on 3D organoids, organs-on-chips, and RNAi-based functional genomics in oncology.
Funding for the work at UBC includes:
Grants from the Leducq Foundation Transatlantic Network of Excellence, C150 Research Chairs Program, Allen Distinguished Investigators award, CIHR, and a UK-Canada Diabetes Research Team Grant.
Link to the Original Paper:
Quintard C, Tubbs E, Jonsson G, Jiao J, Wang J, Werschler N, Laporte C, Pitaval A, Bah TS, Pomeranz G, Bissardon C, Kaal J, Leopoldi A, Long DA, Blandin P, Achard JL, Battail C, Hagelkruys A, Navarro F, Fouillet Y, Penninger JM*, Gidrol X*. A microfluidic platform integrating functional vascularized organoids-on-chip. Nature Communications. 2024 Feb 16:15(1):1452. DOI: 10.1038/s41467-024-45710-4
*co-corresponding authors