Ten LSI researchers were awarded funds in the CIHR Spring 2023 Project Grant competition. Listed below are the LSI-based PIs and a description of the funded projects, including one Priority Announcement and one Bridge grant. In total, these grants were awarded > $8 million from this competition.
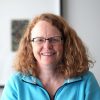
The role of Dystrogylcan and conservation of function in development and maintenance of the peripheral glial sheath
For our nervous system to function each nerve that controls our movement or our response to touch, pain or temperature is bundled by a special cell layer that insulates and protects these nerves. The special cell layer is created by glial cells which surround or ensheath either individual neurons or bundles of neurons. Loss of the insulating layer leads to loss of nervous system function and degeneration or death of the neurons. While much is known about how the insulating layer develops, much less is known on how this layer is maintained as we grow and through adulthood. This is important because as we grow our nerves become longer and larger and the insulating glia need to match this grow. The same is true in adulthood where the glia need to maintain the insulation for decades. To understand how these processes are controlled we use a simple model system, the fruitfly, which has the same programs for the development of glia insulation and also has the same need for maintenance of the glia as the animal grows and in adults. We can use the simple nervous system of the fruit fly and powerful genetic approaches possible in this system to identify and understand how the glial insulation forms, expands and matures and use this knowledge to an understanding of glial insulation in all animals from flies to humans.
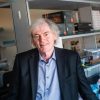
Rational Design of Lipid Nanoparticle Systems for mRNA Delivery
Co-investigator: Eric Jan (LSI)
The COVID-19 pandemic, despite the tremendous burdens it has placed on society, brought to the forefront of medicine a new kind of drug – ribonucleic acid (RNA) medicines. The use of RNA, in particular mRNA molecules that provide instructions to human cells to make a specific protein, as drugs has been an active area of research for some time, but has only recently achieved global recognition with the approval of the COVID-19 mRNA vaccines from Pfizer and Moderna. Such vaccines, and indeed any drug that employs an RNA molecule, require a drug delivery mechanism, both to carry the RNA molecule to our cells and then to release the RNA once safely inside the cell. Lipid nanoparticles (LNP) are nano-sized bubbles that have been engineered to safely and efficiently perform this task. LNP are one of the key innovations that has allowed RNA medicines to gain traction for clinical applications. LNP are composed of multiple types of lipid species. Each component plays a specific function within the LNP. Decades of research have made step-wise improvements to LNP performance, with each iteration providing an LNP formulation that is slightly more effective or safer than its predecessor. As the leading pioneer of LNP technology, Dr. Cullis and his research group continue to make significant advances in their ability to engineer LNP for use in RNA medicines. Recently, Dr. Cullis’ group discovered a structural alteration to LNP that increases the effectiveness of mRNA delivery to cells by as much as 100-fold over previously used formulations. This remarkable discovery is a significant step towards our ability to rationally design better LNP as opposed to simply screening many formulations. Ultimately, rational design will speed up the process of drug development. The purpose of this research program is to better characterize, understand, and apply this ‘next-generation’ LNP formulation in order to enable new and better RNA medicines in the future.
*Dr. Cullis is also co-investigator on a separate awarded grant in this competition, with PIs Dr. Anna Blakney and Dr. Colin Ross, entitled “Development and evaluation of novel nanoparticle formulations for therapeutic genome editing”.
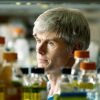
Steroid catabolism in mycobacteria
Mycobacteria cause a number of serious diseases. For example, more people die of tuberculosis (TB) each year than any other infectious disease other than Covid. The bacterium that causes the disease, Mycobacterium tuberculosis (Mtb), infects one third of all people world-wide. With the rise of drug resistant strains and co-infection with HIV, TB remains a global health emergency. In Canada, TB is of particular concern in the First Nations. The treatment of even drug-sensitive TB is arduous, with patients taking a cocktail of toxic chemotherapeutics for six months. Novel therapies are urgently needed to decrease the length, and improve the efficacy, of treatment. A related bacterial strain, Mycobacterium abscessus (Mab), is responsible for pulmonary infections often associated with Cystic Fibrosis (CF). Mab is intrinsically drug resistant, complicating treatment of infections, and the global incidence of Mab infections is rising. Thus, novel therapies are also required to treat Mab infections. We discovered a pathway in Mtb that enables the pathogen to grow on cholesterol during infection. This pathway has become a target for novel drugs to treat TB. We recently demonstrated that Mab has a similar pathway to grow on cholesterol, and that the bacterium appears to also use cholesterol during infection. Nevertheless, there are many aspects of Mtb’s and Mab’s abilities to degrade cholesterol that are not yet known. The overall objective of this project is to better understand Mtb’s and Mab’s cholesterol degradation pathway, including how this pathway works and how it enables these bacteria to be such pernicious pathogens. Improved understanding of the pathway will facilitate the development of novel drugs urgently needed to combat the diseases caused by these pathogens.
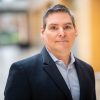
Pre-clinical development of a novel class of broad-spectrum natural product antivirals
Co-investigator: Raymond Andersen (UBC Chemistry)
Emerging human viruses such as SARS-CoV-2 (cause of COVID-19), influenza A virus (acute respiratory infection), and Zika virus (microcephaly and other congenital malformations), have had an enormous impact on human health. The World Health Organization (WHO) has declared these three to be Public Health Emergencies of International Concern (PHEICs). Although vaccines have greatly limited COVID-19 and Influenza-related hospitalizations and deaths, these viruses are continually changing, rendering vaccines less effective over time. What’s more, no vaccine is available yet for preventing Zika virus infection. Importantly, no broad-spectrum antiviral drugs are available against these three viruses despite the fact that each virus was declared a PHEIC [influenza A pandemic (2009); Zika virus epidemic (2016); COVID-19 pandemic (2019)]. Our research team has discovered an exciting drug that can block infections in human cells caused by SARS-CoV-2, influenza A, and Zika, which we call a broadly acting antiviral. The drug, called cladoniamide A (CA), is a natural product (NP) discovered in British Columbia that blocks a human protein complex called vacuolar ATPase that helps human viruses get into our cells. NPs as drugs have fewer side effects and a faster approval process than chemically engineered drugs, a great advantage in dealing with a pandemic. In this research proposal, we plan to further improve the pharmaceutical and antiviral properties of CA and develop a new class of nature-based broad-spectrum medicines against SARS-CoV-2, influenza A, and Zika viruses. We anticipate that this research will further develop cladoniamide A and its derivatives so they can be further tested in clinical trials. In the future, this will lead to new and exciting broad-spectrum possibilities for treating other human viral diseases of global health concern.
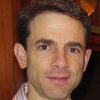
Dissecting the interplay between histone marks in directing transcription in pluripotent stem and neural precursor cells
Co-investigator: Sanne Janssen (LSI)
Each cell in an individual has the same genetic code, our DNA. Yet, our body consists of many different cell types. This is due the ability of our cells to turn genes on and off, depending on which genes are needed within that specific cell type. DNA in our cells is wrapped around protein scaffolds called histones. Chemical modifications to such histones act like levers that a cell uses to control whether to turn a gene on or off. We call the study of these modifications Epigenetics. Epigenetic enzymes modify specific amino acids on histones. These modifications can enable or disrupt the activity of other epigenetic enzymes, resulting in specific modifications at specific locations along the DNA. The balance between different histone modifications is disturbed when a specific epigenetic enzyme is defective, ie mutated, as observed in neurodevelopmental disorders and cancers with mutations in the genews encoding these proteins. When histones are modified at abnormal locations along the DNA it leads to malfunctioning gene control. It is unclear how disrupting the balance between histone modifications leads to misguided control of genes. To gain insight into this, we will first use gene editing to delete specific epigenetic enzymes. Then, we will examine the effect of such deletion on histone modifications and gene control. This allows us to show how disrupting the balance between histone modifications results in abnormal gene control and will shed light on how defective epigenetic enzymes can contribute to disease. These discoveries will assist in the development of new treatments for the neurodevelopmental disorders and cancers in which these epigenetic enzymes are mutated.
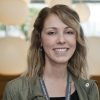
The role of microglia in mediating sex-specific neurodevelopmental responses to maternal stress
The prevalence of neurodevelopmental disorders such as autism spectrum disorder (ASD), attention-deficit/hyperactivity disorder (ADHD), and various other intellectual and learning disabilities has dramatically increased in Canada and worldwide over the last 2 decades. While understanding why neurodevelopmental disorders are on the rise is complex-as genetic disruptions and environmental challenges have both been shown to contribute to abnormal brain function and behavioural impairments-we are becoming increasingly aware that the fetus is critically sensitive to early life exposures and disrupting the intrauterine environment may play a larger role in the rise in neurodevelopmental disorders than once thought. For example, maternal insults and exposures have been shown to disrupt fetal neurodevelopment, resulting in lifelong neuropsychiatric consequences. A common feature reported across various studies relates to disruptions of the immune system, suggesting that immune cells within the developing fetal brain may sense changes in their surroundings and respond by altering brain development. Although we have made important advancements in our understanding of the genetic contribution to neurodevelopmental disorders, our understanding of how the intrauterine environment impacts fetal brain development has lagged behind-especially as it relates to maternal mental health and the involvement of maternal stress in fetal brain disruptions. To address this gap in our knowledge, my laboratory will study how microglia-the immune cells of the brain-sense maternal stress and transmit this information to nearby neural cells, thereby altering brain development in the fetus. We predict that microglia may be an underappreciated fetal brain sensor-providing key linkages into how the maternal environment influences the developing brain. This is important as it may provide new avenues for managing and treating neurodevelopmental disorders.
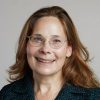
Reinforcing the battle at the bacterial cell wall: Structure-guided characterization and inhibition of beta-lactam antibiotic resistance signalling mechanisms
Antibiotic resistance is a major global health concern. The mortality and health care costs associated with spread and persistence of antibiotic resistant strains of bacteria, many of which are widely disseminated in hospitals and communities in Canada, the US and world wide, are increasingly dire. At the same time we have few new therapies in the pipeline to treat these increasingly resistant superbugs now and in the future. The protein machinery that create the bacterial cell wall represent one of the greatest drug targets in history, as validated by the amazing success of beta-lactam antibiotics such as penicillins and cephalosporins in near eight decades of treating life threatening infections. Still by far the most common antibiotic class prescribed for reasons of economy, efficacy and side effects, there is still considerable interest in preserving their action by creating cocktails of beta-lactam antibiotic compounds with inhibitors of drug resistance mechanisms inherent to many infectious bacterial strains. Using our state of the art biophysical toolbox we will determine atomic level pictures of central proteins and protein complexes that mediate beta-lactam antibiotic resistance. Coupled with complementary biochemical and cellular microbiology analysis, these first to field molecular “blueprints” will inform design of new inhibitors and therapies to target infections from notoriously drug resistant, and often hospital acquired superbugs such as Staphylococus aureus, Clostridium difficile, Mycobacterium tuberculosis and Pseudomonas aeruginosa.
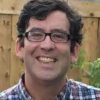
Mechanisms of regulation of Cell-Matrix adhesion in development and disease
Integrins are the main molecules in the human body that link cells to the extracellular matrix (ECM), a mixture of complex sugars and proteins that forms the base upon which tissues are built. Integrins are very important for many processes and disruptions in integrin function are associated with a large array of disease processes in humans. We are interested in understanding the mechanisms by which integrin carry out their diverse functions as this will give us crucial insight into many disease processes. To understand how integrins carry out so many diverse functions we are focusing on how they are regulated, that is, how they are switched on and off at certain times. Our proposal presents experiments that seek to understand how integrins are regulated and how this regulation contributes to tissue formation and repair. We propose to use flies as a pipeline to quickly identify, by employing genetic approaches, regulatory mechanisms that control integrin-based adhesion to the ECM and to uncover how these mechanisms are used during tissue development. Next we use our analysis pipeline to characterise mutations that have been identified in human patients to cause various rare genetic diseases. Although we know what these mutations are, clinicians currently do not understand how these mutations function to give rise to disease. We will be able to provide a detailed analysis of the effects of these mutations on integrin regulation and establish a simple animal model to study these disease causing mutations further. Overall, results of this study will shed important light on how integrins are controlled during tissue formation and tissue maintenance, and also provide insight into how dysregulation of integrin function leads to disease.
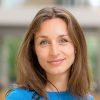
Impact of loss of commensal bacteria on enteric infection
Priority Announcement
Human health is intimately connected to our microbiota, a unique, constantly evolving consortium of trillions of bacteria that live in and on our bodies. Gut microbes produce compounds that are absorbed into our blood, providing nourishment, and affecting diverse functions such as digestion, immunity, neurodevelopment, as well as colonization resistance against invading and potentially harmful microorganisms, or “pathogens”. Antibiotics have been shown to disrupt the gut microbiota and decrease its ability to protect the host against infection. Beyond antibiotics, it is not known what other microbiota disruptions may reduce colonization resistance. My lab has been investigating how the loss of specific microbiota members affects infection by gut pathogens. In this project we will use a combination of cutting-edge experimental and computational techniques to study pathogen infection after bacterial depletion. This work will also investigate microbiota therapies that can counteract the loss of species and increase microbiota diversity to promote resilience to infection, while identifying the mechanisms involved in microbiota resilience to pathogen invasion. This research will lead to a deeper understanding of pathogen infections and inform the development of new probiotic therapies that will benefit the health of millions of Canadians.
*Dr. Tropini is also co-investigator on a separate awarded grant in this competition, with PI Dr. Annie Ciernia (Djavad Mowafaghian Centre for Brain Health), entitled “Metabolite Control of Microbiome-Microglia Communication in Inflammatory Bowel Disease”
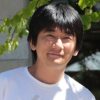
Elucidating the novel role of Calcium/calmodulin-dependent protein kinase II (CaMKII) in presynaptic assembly
Bridge Grant
Our brain consists of billions of nerve cells that communicate with each other to conduct complex tasks. The nerve cells use a cellular interface called synapse to send and receive information. Synapse contains many different types of proteins, each of which plays different roles for a synapse to function normally. If the synaptic structure is abnormal, nerve cells cannot communicate correctly, and brain function will be severely affected. Indeed, abnormal structure and function of synapses are often observed in the brains of patients with various neurological disorders, including intellectual disability. Currently, few therapeutics are available for these neurological disorders, partly because of the lack of understanding of how synapse is assembled and how its abnormality leads to these diseases. Therefore, we urgently need to increase our knowledge of basic mechanisms of synapse formation. We tackle this important issue by using nematode (C. elegans) as a genetic model organism. C. elegans has a simple nervous system, yet its nerve cells and synapses are highly similar to those of humans. Historically, many studies have proven that the discoveries made in C. elegans nerve cells and synapses can be directly transferred to understand how our nerve cells and synapses develop. Specifically, we focus on studying a gene called Calcium/calmodulin-dependent protein kinase II (CaMKII), whose mutations in humans are highly associated with various neurological disorders including intellectual disabilities. Our current data strongly suggests that CaMKII plays a crucial role in assembling multiple proteins into the functional synapses. Uncovering the novel functions of CaMKII in synapse formation will help us better understand how our brain develops, and how mutations in CaMKII cause various neurological diseases, which are the first steps toward the future development of the therapeutics.